The Ocean Impact Project
Supporting Game-Changing Ocean Regeneration Projects
Sustainable ocean ecosystems are essential for all life, even on land. Our oceans produce half of the oxygen we breathe, they represent a large part of our diet, and they offer perhaps our greatest opportunity to reverse climate change.
Without healthy ocean ecosystems much of life on earth will be threatened, and many of these habitats are on the verge of collapse[3].
We’ve explored these issues with the same level of rigour and analysis that we review portfolio companies. We want to share our research, but most importantly, we’re offering vital financial support to some of the most exciting solutions. We invite you to join us on our mission to help heal our oceans.
Blue Oceans Capital devotes 50% of management fee profits to help find solutions to the immense problem of ocean exploitation.
We’ve identified some of the leading research teams who have active projects with huge potential to reverse the damage being done to our oceans. Plus, we’ve employed economic ecologist, Anya Phelan, to guide our strategy and to advise on project selection and impact measurement.
Join Blue Oceans Capital, and help these organisations to grow.
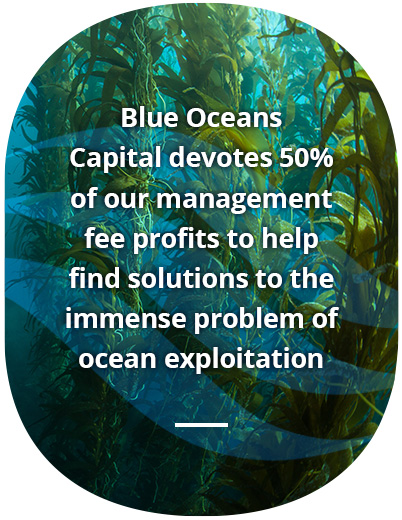
Our Beneficiaries
Climate Foundation
Fighting climate change through producing seaweed on an industrial scale, and driving large-scale carbon drawdown.
GreenWave
Developing regenerative ocean farming practices for seaweed and shellfish, while also sequestering carbon, rebuilding reef ecosystems and reducing catch pressure on wild species.
University of Tasmania
Recognised as a world leader in ocean sustainability research, and pioneering new climate change solutions.
Seaweed – A possible solution
The oceans need to be cooler and to hold much less carbon. To do this humanity must reverse its carbon emissions as soon as possible. Transitioning towards net zero economies is both essential and urgent if we are to have even a chance at limiting global warming to below 2 degrees celsius[4].
However, achieving global net zero will not go far enough. We will still be left with the task of drawing down an additional 100 – 900 gigatons of CO2 from the atmosphere by 2100[5]. What is required is industrial scale solutions to draw down carbon on a gigatonne scale.
Seaweed is the unlikely organism that has huge potential as a solution to the greatest challenge currently facing humanity.
Oxygen generating system under threat
Phytoplankton form the base of the marine food web and are an essential organism for the survival of life on Earth. Every second breath we take is oxygen produced mostly by phytoplankton[6]. These microscopic organisms are responsible for cycling most of the globe’s carbon dioxide between the oceans and the atmosphere[7].
Phytoplankton rely on upwelling for nutrients however evidence shows significant disruption to phytoplankton populations through the reduction of upwelling, and associated nutrient loss in many open oceans[8]. That loss of upwelling stems from increasing water temperatures due to climate change[9]. Global Oceans have continuously warmed since 1970 and have taken up more than 90% of the excess heat in the climate system[10].
The world’s oceans have acted as a buffer to rising CO2 levels in the atmosphere by absorbing excess CO2 into the water. Around 25% of the CO2 released by human activity is taken up by the oceans[11]. However that absorption has come at the cost of increased ocean acidification and a loss of oxygen from the surface down to a depth of 1000m[12].
Ocean deoxygenation is another side effect of climate change that is creating significant damage[13], particularly along coastlines which are vulnerable to hypoxic events[14].
SOLUTION:
Seaweed agriculture provides oxygen-rich habitats that give refuge to marine organisms [15][16] offering a real environmental benefit by near-shore seaweed farms.
Seaweed farms provide habitat for herbaceous fish species that support higher order species in the food chain. These dense habitats support commercial fishing on the seaweed farms that reduces the pressure on wild catch. Research by the Wageningen University in the Netherlands has found that 180,000 square kilometers of seaweed farms could produce enough protein to feed the entire world[17]. Conducting agriculture in this way has the potential to substantially reduce the pressure humankind is exerting on natural ecosystems across land and water.
Climate change is killing sea-life, and destroying habitats
Coral reefs are essential habitat for the maintenance of marine ecosystems. Around 25% of the ocean’s fish rely on healthy coral reefs with many species depending on coral habitats for shelter, food and reproduction[18]. However increasing acidification, caused by excess CO2 in the oceans, is leading to dissolving coral reefs[19]. One study by Nature Journal finds that 25% of 36 reefs studied around the world were significantly degraded and that reefs may become net-dissolving between 2031 and 2082[20].
Climate change is also heating up our oceans. Rapidly accelerating sea surface temperatures are increasing the frequency and intensity of coral bleaching events globally [21][22][23]. Bleaching events are deadly to coral, decreasing the amount of coral cover and devastating essential coral-reef-fish communities that has flow on effects up through the food chain[24][25].
Like corals, seaweeds are key habitat structures that harbour magnificent biodiversity. In many parts of the world seaweed forests are declining due to climate change, overfishing and wild harvesting[26][27]. Climate change has a significant indirect effect through sea urchins.
Sea urchins are major grazers of kelp world wide preferring to sit immobile feeding off drift kelp that falls off in giant stands of healthy kelp. However increasing storm intensity associated with climate change creates large scale disturbances that remove vast amounts of kelp forest. Sea urchins mobilise in these conditions grazing on young kelp. They also climb and feed on adult kelp, removing kelp strands and preventing the reestablishment of the forest[28] after extreme weather events, which is leading to barren seascapes.
This scenario has played out in the California Current Large Marine Ecosystem where bull kelp has lost over 90% of its mass along the coast line[29]
SOLUTION:
Seaweed is a remarkable macroalgae with numerous benefits. As the fastest growing plant in the world, 100m2 of seaweed can capture 16t of carbon per year[30]. Many have advocated for large scale seaweed harvesting as one of the most promising carbon drawdown methods currently available[31][32]. As seaweed draws out carbon dioxide it de-acidifies the water increasing the carbon absorption capacity of the oceans[33].
Plastics are suffocating the ocean, and poisoning our food
The rise in ocean plastic pollution is increasing exponentially worldwide[34]. Research by Ostle, et al. (2019), published in Nature Journal reveals the extent of macroplastic accumulation as presented below in figure 1.
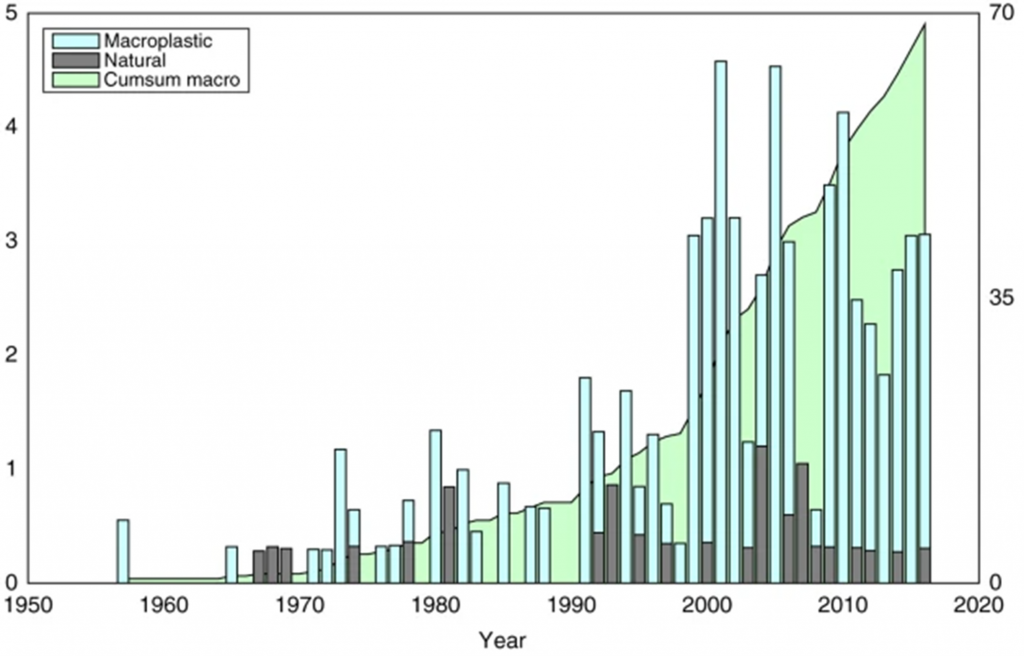
Figure 1. Cumulative Sum of Macroplastics.
Light blue bars = annual counts of macroplastic, grey bars = natural items and green = cumulative sum of total counts of macroplastics plotted on the second y-axis[35].
Plastics are wreaking havoc on our oceans. The most common documented impact stems from entanglement and ingestion of macroplastic debris. Studies have shown that the portion of mammals, turtles and bird species that ingest plastic are 40%, 100% and 46% respectively and that become entangled in it are 46%, 100%, and 26% respectively. Each successive review finds more species are being affected by macroplastic debris[36], some with secondary effects.
For example, Zooplankton consumption of microplastic could decrease water column oxygen by 10% in the North Pacific and accelerate global oxygen loss by an extra 0.2 – 0.5%[37].
Toxic substances can be released upon ingestion and accumulate in fatty tissues. Once in the food chain these microplastics find their way into humans witch could potentially be quite harmful to our health[38]. Ocean plastics are harmful to economies as well. Research by Beaumont et al., has discovered that marine plastics come at a cost of between $3300 to $3300 USD per tonne when considering negative effects on ocean based services[39].
SOLUTION:
Seaweed bioplastics are a viable substitute for fossil fuel plastics and other biomass plastics as they are 100% biodegradable[40], do not require pesticide or land use, grow rapidly and are easy to harvest[41]. The future success of seaweed bioplastics will be dependent on a reliable, industrial scale supply of seaweed feedstock at a price competitive with oil and thus first dependent on the development of seaweed farms.
Seaweed is just one part of the solution
Seaweed farming will not be a silver bullet to our environmental problems but likely part of a much broader solution. More work needs to be done in researching the carbon sequestration potential of seaweed[42]. Prototypes of large scale ocean systems need to be built, studied and iterated. Noting of course, an area 4 times the size of Australia would be required just to absorb the planets current annual CO2 emissions[43], so it’s no silver bullet.
We recognise that seaweed farms could be an essential part of a much broader strategy on carbon drawdown, while also significantly contributing to the sustainability of ocean ecosystems.
References:
- Special Report on the Ocean and Cryosphere in a Changing Climate — . (2021). Retrieved 29 July 2021, from https://www.ipcc.ch/srocc/
- The World’s Oceans Are in Danger, Major Climate Change Report Warns (Published 2019). (2019). Retrieved 2 August 2021, from: https://www.nytimes.com/2019/09/25/climate/climate-change-oceans-united-nations.html
- Special Report on the Ocean and Cryosphere in a Changing Climate — . (2021). Retrieved 29 July 2021, from https://www.ipcc.ch/srocc/
- van Soest, H.L., den Elzen, M.G.J. & van Vuuren, D.P. Net-zero emission targets for major emitting countries consistent with the Paris Agreement. Nat Commun 12, 2140 (2021). https://doi.org/10.1038/s41467-021-22294-x
- Bach, L.T., Tamsitt, V., Gower, J. et al. Testing the climate intervention potential of ocean afforestation using the Great Atlantic Sargassum Belt. Nat Commun 12, 2556 (2021). https://doi.org/10.1038/s41467-021-22837-2
- How much oxygen comes from the ocean?. (2021). Retrieved 2 August 2021, from https://oceanservice.noaa.gov/facts/ocean-oxygen.html
- Society, N. (2014). Plankton Revealed. Retrieved 27 July 2021, from https://www.nationalgeographic.org/media/plankton-revealed/#:~:text=Through%20photosynt
- Boyce, Daniel & Worm, Boris. (2015). Patterns and ecological implications of historical marine phytoplankton change. Marine Ecology Progress Series. 534. 10.3354/meps11411.
- Osman, M.B., Das, S.B., Trusel, L.D. et al. Industrial-era decline in subarctic Atlantic productivity. Nature 569, 551–555 (2019). https://doi.org/10.1038/s41586-019-1181-8
- Climate Change: Ocean Heat Content | NOAA Climate.gov. (2021). Retrieved 2 August 2021, from https://www.climate.gov/news-features/understanding-climate/climate-change-ocean-heat-content
- Ocean Acidification. (2018). Retrieved 2 August 2021, from https://ocean.si.edu/ocean-life/invertebrates/ocean-acidification
- Ocean deoxygenation. (2019). Retrieved 2 August 2021, from www.iucn.org/resources/issues-briefs/ocean-deoxygenation
- Keeling, R. F., Körtzinger, A., and Gruber, N. (2010). Ocean deoxygenation in a warming world. Mar. Sci. 2, 199–229. doi: 10.1146/annurev.marine.010908.163855
- Diaz, R. J., and Rosenberg, R. (2008). Spreading dead zones and consequences for marine ecosystems. Science 321, 926–929. doi: 10.1126/science.1156401
- Duarte, C. M., Wu, J., Xiao, X., Bruhn, A. and Krause-Jensen, D.Duarte, C., Wu, J., Xiao, X., Bruhn, A., & Krause-Jensen, D. (2017). Can Seaweed Farming Play a Role in Climate Change Mitigation and Adaptation?. Frontiers In Marine Science, 4. doi: 10.3389/fmars.2017.00100
- Radulovich, R., Umanzor, S., Cabrera, R., and Mata, R. (2015). Tropical seaweeds for human food, their cultivation and its effect on biodiversity enrichment. Aquaculture 436, 40–46. doi: 10.1016/j.aquaculture.2014.10.032
- Growing seaweed can solve acidification. (2021). Retrieved 3 August 2021, from https://phys.org/news/2010-12-seaweed-acidification.html
- Coral reef ecosystems | National Oceanic and Atmospheric Administration. (2021). Retrieved 27 July 2021, from https://www.noaa.gov/education/resource-collections/marine-life/coral-reef-ecosystems
- Eyre, B., Cyronak, T., Drupp, P., De Carlo, E., Sachs, J., & Andersson, A. (2018). Coral reefs will transition to net dissolving before end of century. Science, 359(6378), 908-911. doi: 10.1126/science.aao1118
- Davis, K.L., Colefax, A.P., Tucker, J.P. et al. Global coral reef ecosystems exhibit declining calcification and increasing primary productivity. Commun Earth Environ 2, 105 (2021). https://doi.org/10.1038/s43247-021-00168-w
- Hoegh-Guldberg, O. et al. Coral reefs under rapid climate change and ocean acidification. Science 318, 1737–1742 (2007).
- Hughes, T. P. et al. Spatial and temporal patterns of mass bleaching of corals in the Anthropocene. Science 359, 80–83 (2018).
- Pandolfi, J. M., Connolly, S. R., Marshall, D. J. & Cohen, A. L. Projecting coral reef futures under global warming and ocean acidification. Science 333, 418–422 (2011).
- Loya, Y. et al. Coral bleaching: the winners and the losers. Ecol. Lett. 4, 122–131 (2001).
- Stuart-Smith, R. D., Brown, C. J., Ceccarelli, D. M. & Edgar, G. J. Ecosystem restructuring along the Great Barrier Reef following mass coral bleaching. Nature 560, 92–96 (2018).
- Murie, K.A., Bourdeau, P.E. Energetic context determines the effects of multiple upwelling-associated stressors on sea urchin performance. Sci Rep 11, 11313 (2021). https://doi.org/10.1038/s41598-021-90608-6
- Krumhansl, K. A. et al. Global patterns of kelp forest change over the past half-century. Proc. Natl. Acad. Sci. USA 113, 13785–13790 (2016).
- Pearse, J. S. Ecological role of purple sea urchins. Science 31, 940–941 (2006).
- Watanabe, J. M. & Harrold, C. Destructive grazing by sea urchins Strongylocentrotus spp. in a central California kelp forest: potential roles of recruitment, depth, and predation. Mar. Ecol. Prog. Ser. 71, 125–141 (1991).
- Flannery, T. (2017). “Can seaweed save the world?”
- How farming giant seaweed can feed fish and fix the climate. (2017). Retrieved 2 August 2021, from https://theconversation.com/how-farming-giant-seaweed-can-feed-fish-and-fix-the-climate-81761
- Sondak, C.F.A., Ang, P.O., Beardall, J. et al. Carbon dioxide mitigation potential of seaweed aquaculture beds (SABs). J Appl Phycol 29, 2363–2373 (2017). https://doi.org/10.1007/s10811-016-1022-1
- How farming giant seaweed can feed fish and fix the climate. (2017). Retrieved 29 July 2021, from https://theconversation.com/how-farming-giant-seaweed-can-feed-fish-and-fix-the-climate-81761
- Worm, B., Lotze, H. K., Jubinville, I., Wilcox, C. & Jambeck, J. Plastic as a Persistent Marine Pollutant. Annu. Rev. Environ. Resour. 42, 1–26 (2017).
- Ostle, C., Thompson, R.C., Broughton, D. et al. The rise in ocean plastics evidenced from a 60-year time series. Nat Commun 10, 1622 (2019). https://doi.org/10.1038/s41467-019-09506-1
- Worm, B., Lotze, H. K., Jubinville, I., Wilcox, C. & Jambeck, J. Plastic as a Persistent Marine Pollutant. Annu. Rev. Environ. Resour. 42, 1–26 (2017).
- Kvale, K., Prowe, A., Chien, C., Landolfi, A., & Oschlies, A. (2021). Zooplankton grazing of microplastic can accelerate global loss of ocean oxygen. Nature Communications, 12(1). doi: 10.1038/s41467-021-22554-w
- Lim, X. (2021). Microplastics are everywhere — but are they harmful?. Retrieved 29 July 2021, from https://www.nature.com/articles/d41586-021-01143-3
- Beaumont, N., Aanesen, M., Austen, M., Börger, T., Clark, J., & Cole, M. et al. (2019). Global ecological, social and economic impacts of marine plastic. Marine Pollution Bulletin, 142, 189-195. doi: 10.1016/j.marpolbul.2019.03.022
- Seaweed Product R&D — Australian Seaweed Institute. (2021). Retrieved 2 August 2021, from https://www.australianseaweedinstitute.com.au/about-us#:~:text=With%20huge%20potential%20
- Lim, C., Yusoff, S., Ng, C., Lim, P., & Ching, Y. (2021). Bioplastic made from seaweed polysaccharides with green production methods. Journal Of Environmental Chemical Engineering, 9(5), 105895. doi: 10.1016/j.jece.2021.105895
- Bach, L.T., Tamsitt, V., Gower, J. et al. Testing the climate intervention potential of ocean afforestation using the Great Atlantic Sargassum Belt. Nat Commun 12, 2556 (2021). https://doi.org/10.1038/s41467-021-22837-2
- N’Yeurt, A. D. R., Chynoweth, D. P., Capron, M. E., Stewart, J. R. & Hasan, M. A. Negative carbon via ocean afforestation. Process Saf. Environ. Prot. 90, 467–474 (2012).